- GD&T
GENERAL DIMENSIONING- International Paper Size Standards
- Technical Drawing Styles
- ISO And ANSI Projections
- ANSI Technical Drawing Views
- Technical Drawing Dimesioning Types
- ANSI and ISO Geometric Tolerancing Symbols
- Geometric Tolerancing Reading
- Taylor Principle Rule#1
- Form Tolerances
- Profile Tolerances
- Orientation Tolerances
- Location Tolerances
- Runout Tolerances
- TOLERANCES
ANSI AND ISO- Tolerancing and Engineering Standards
- Hole and Shaft Basis Limits And Fits
- ISO International System For Limits And Fits
- International Tolerance Grade (IT)
- Fundamental Deviations For Hole and Shaft Basis
- ISO Tolerance Band IT01-IT16
- Calculation Of International Tolerance
- Calculation of Upper and Lower Deviation For Shaft
- Calculation of Upper and Lower Deviation For Holes
- ISO Shaft Tolerances (3mm-400mm)
- ISO Shaft Tolerances (400mm-3150mm)
- ISO Hole Tolerances (3mm-400mm)
- ISO Hole Tolerances (400mm-3150mm)
- ANSI Standard Limits and Fits
- METAL CUTTING TECHNOLOGIES
- Terms and Definitions of the Cutting Tools
- Cutting Tool Materials
- Selection of Carbide to machine the work-part
- Identification System For Indexable Inserts
- Work-Part Materials
- Machinability and the specific cutting force
- Machinability of the Certain Material Evaluations
- Cutting Forces and Chip Formations
Iron-Nickel-Base Superalloys
Iron-nickel-base superalloys are similar to wrought austenitic stainless steels, except for the addition of γ´ strengthening agent.
They have the lowest elevated temperature strength among the three groups of superalloys, and are generally used in the wrought condition
in gas turbine disks and blades. Most wrought alloys contain high levels of chromium, which provide corrosion resistance.
They owe their high-temperature strength to solid solution hardening (hardening produced by solute atoms dissolved in the alloy matrix)
or precipitation hardening (hardening produced by precipitate particles). Alloys such as Haynes 556 and 19-9 DL are solid solution
strengthened with molybdenum, tungsten, titanium, and niobium. Alloys such as A286 and Incoloy 909 are precipitation hardened.
The most common precipitates are γ´, (Ni3 [Al, Ti]) (e.g., A286), and γ˝, (Ni3Nb) (e.g., Incoloy 909).
Another group of iron-nickel-base alloys contains high carbon content and is strengthened by carbides, nitrides,
and solid solution strengthening. A group of alloys, based on Fe-Ni-Co and strengthened by γ’, combines high
strength with a low thermal expansion coefficient (e.g., Incoloy 903, 907, and 909) and finds application in shafts,
rings, and casings for gas turbines. Material Characteristics of Iron-Nickel-Base Superalloys; Relatively poor tool life.
Small depths of cut are difficult. Rapid workhardening. Usually abrasive rather than hard. Tough and stringy chips.
Troubleshooting when cutting Iron-Nickel-Base Superalloys;
Problem of Depth-of-cut notch: Solution for it; Increase toolholder lead angle.
Use tougher grade, Use a 0,63mm/.025" or greater depth of cut. Feed greater than 0,12mm/.005 IPR.
Increase coolant concentration. Vary depth of cut. Depth of cut should be greater than the workhardened
layer resulting from the previous cut (greater than 0,12mm/.005").
Problem of Built-up edge: Increase speed. Use positive rake, sharp PVD coated grades or Use ceramic grades.
Problem of Torn Workpiece surface finish: Solution for it; Increase speed. Reduce feed. Increase coolant concentration. Use a GG-FS, GT-HP, or GT-LF geometry.
Problem of Workpiece glazing: Solution for it; Increase depth of cut. Increase feed rate and decrease speed. Reduce insert nose radius size. Use a GG-FS, GT-HP, or GT-LF geometry. Use PVD grade.
Titanium and Titanium Based Alloys
Alpha (α) Alloys; Pure titanium and titanium alloyed with α stabilizers, such as tin and aluminum
(e.g., Ti5Al2.5Sn), are classified as α alloys. They are non-heat treatable and are generally weldable.
They have low to medium tensile strength, good notch toughness, and excellent mechanical properties at cryogenic temperatures.
Beta (β) Alloys; Beta (β) alloys contain transition metals, such as V, Nb, Ta, and Mo, that stabilize the β phase. Examples of commercial β alloys include Ti11.5Mo6Zr4.5Sn, Ti15V3Cr3Al3Sn, and Ti5553. Beta alloys are readily heat-treatable, generally weldable, and have high strengths. Excellent formability can be expected in the solution treated condition. However, β alloys are prone to ductile-brittle transition and thus are unsuitable for cryogenic applications. Beta alloys have a good combination of properties for sheet, heavy sections, fasteners, and spring applications.
Titanium Alloys; Pure titanium (Ti) undergoes a crystallographic transformation, from hexagonal close packed, hcp (alpha, α) to body-centered cubic, bcc (beta, β) structure as its temperature is raised through 1620 °F / 882°C. Alloying elements, such as tin (Sn), when dissolved in titanium, do not change the transformation temperature, but elements such as aluminum (Al) and oxygen (O) cause it to increase. Such elements are called “α stabilizers.” Elements that decrease the phase-transformation temperature are called “β stabilizers.” They are generally transition metals. Commercial titanium alloys are thus classified as “α,” “α-β,” and “β.” The α-β alloys may also include “near α” and “near β” alloys depending on their composition.
Alpha-Beta (α-β) Alloys; These alloys feature both α and β phases and contain both α and β stabilizers. The simplest and most popular alloy in this group is Ti6Al4V, which is primarily used in the aerospace industry. Alloys in this category are easily formable and exhibit high room-temperature strength and moderate high-temperature strength. The properties of these alloys can be altered through heat treatment.
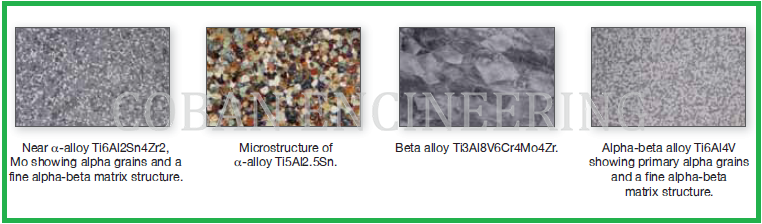
Machinability of Titanium Alloys;
Titanium is one of the fastest growing materials used in aerospace applications.
The prime rationale for designers to chose titanium in their designs is its relative low mass for a given strength
level and its relative resistance to high temperature. Titanium has long been used in aircraft engine front sections
and will continue to be used there for the foreseeable future. In fact, due to its properties, titanium alloys are
becoming more prevalent than ever before in structural and landing gear components. Machining of titanium alloys
is as demanding as the cutting of other high-temperature materials. Titanium components are machined in
the forged condition and often require removal of up to 90% of the weight of the workpiece. The high-chemical
reactivity of titanium alloys causes the chip to weld to the tool, leading to cratering and premature tool failure.
The low thermal conductivity of these materials does not allow the heat generated during machining to dissipate
from the tool edge. This causes high tool tip temperatures and excessive tool deformation and wear. Titanium
alloys retain strength at high temperatures and exhibit low thermal conductivity. This distinctive property
does not allow heat generated during machining to dissipate from the tool edge, causing high tool tip temperatures
and excessive plastic deformation wear leading to higher cutting forces. The high work-hardening tendency of
titanium alloys can also contribute to the high cutting forces and temperatures that may lead to depth-of-cut
notching. In addition, the Chip-Tool contact area is relatively small, resulting in large stress concentration
due to these higher cutting forces and temperatures resulting in premature failure of the cutting tool.
The low Modulus of Elasticity (Young’s Modulus) of these materials causes greater workpiece spring
back and deflection of thin-walled structures resulting in too l vibration, chatter and poor surface finish.
Alpha (α) titanium alloys (Ti5Al2.5Sn, Ti8Al1Mo1V, etc.) have relatively low tensile strengths (σT)
and produce relatively lower cutting forces in comparison to that generated during machining of alpha-beta (α−β)
alloys (Ti6Al4V) and even lower as compared to beta (β) alloys (Ti10V2Fe3Al) and near beta (β) alloys (Ti5553).
A generous quantity of coolant with appropriate concentration should be used to minimize high tool tip temperatures
and rapid tool wear. Positive-rake sharp tools will reduce cutting forces and temperatures and minimize part deflection.
Material Characteristics Titanium and Titanium Based Alloys; Relatively poor tool life, even at low cutting speeds. High chemical reactivity causes chips to gall and weld to cutting edges. Low thermal conductivity increases cutting temperatures. Usually produces abrasive, tough, and stringy chips. Take precautionary measures when machining a reactive (combustible) metal. Low elastic modulus easily causes deflection of workpiece. Easy work hardening.
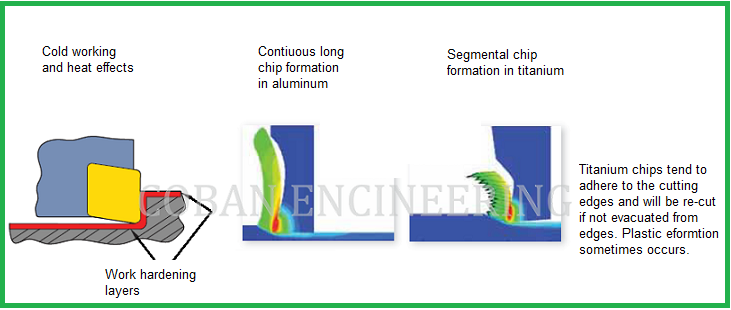
Troubleshooting of Titanium and Titanium Based Alloys;
Problem of Depth-of-cut notch: Solution for it; Avoid built-up edge. Increase the tool lead angle. Use tougher grades
in -UP, -MP, or -RP geometries for interrupted cutting or in “S” edge geometries for Milling. Maintain speed
and decrease feed rate simultaneously. Use MG-MS geometry in place of GP. Ensure proper insert seating. Increase coolant
concentration. Depth of cut should be greater than the work-hardened layer resulting from the previous cut (greater than 0,12mm/.005").
Use strongest insert shape possible. Program a ramp to vary depth of cut.
Problem of Built-up edge: Solution for it; Maintain sharp or lightly honed cutting edges. Use ground periphery inserts and index often. Use GG-FS or GT-LF geometry in PVD. Increase speed. Increase feed. Increase coolant concentration.
Problem of Torn workpiece surface finish: Solution for it; Increase feed and reduce speed. Use positive rake, sharp PVD-coated grades. Increase speed. Increase coolant concentration.
Problem of Workpiece glazing: Solution for it; Increase depth of cut. Reduce nose radius. Index insert to sharp edge. Do not dwell in the cut.
Copyright ©2010-2023 Coban Engineering.All Rights Reserved.